Bio-materials typically have an intrinsically low electrical conductivity, and so the availability of a bio-material with extraordinary electrical properties has great potential for new applications in bio-electronics. This prospect of technological application however requires a deeper understanding of the mechanism of electron transport as well as the structure and composition of the conductive fibers in cable bacteria.*
Filamentous cable bacteria display long-range electron transport, generating electrical currents over centimeter distances through a highly ordered network of fibers embedded in their cell envelope. The conductivity of these periplasmic wires is exceptionally high for a biological material, but their chemical structure and underlying electron transport mechanism remain unresolved.*
In their article “Efficient long-range conduction in cable bacteria through nickel protein wires” Henricus T. S. Boschker, Perran L. M. Cook, Lubos Polerecky, Raghavendran Thiruvallur Eachambadi, Helena Lozano, Silvia Hidalgo-Martinez, Dmitry Khalenkow, Valentina Spampinato, Nathalie Claes, Paromita Kundu, Da Wang, Sara Bals, Karina K. Sand, Francesca Cavezza, Tom Hauffman, Jesper Tataru Bjerg, Andre G. Skirtach, Kamila Kochan, Merrilyn McKee, Bayden Wood, Diana Bedolla, Alessandra Gianoncelli, Nicole M. J. Geerlings, Nani Van Gerven, Han Remaut, Jeanine S. Geelhoed, Ruben Millan-Solsona, Laura Fumagalli, Lars Peter Nielsen, Alexis Franquet, Jean V. Manca, Gabriel Gomila and Filip J. R. Meysman combine high-resolution microscopy, spectroscopy, and chemical imaging on individual cable bacterium filaments to demonstrate that the periplasmic wires consist of a conductive protein core surrounded by an insulating protein shell layer.*
The core proteins contain a sulfur-ligated nickel cofactor, and conductivity decreases when nickel is oxidized or selectively removed. The involvement of nickel as the active metal in biological conduction is remarkable, and suggests a hitherto unknown form of electron transport that enables efficient conduction in centimeter-long protein structures.*
NANOSENSORS wear-resistant conductive Platinum Silicide AFM probes of the PtSi-CONT type were used for the Scanning dielectric microscopy (SDM) described in the article.
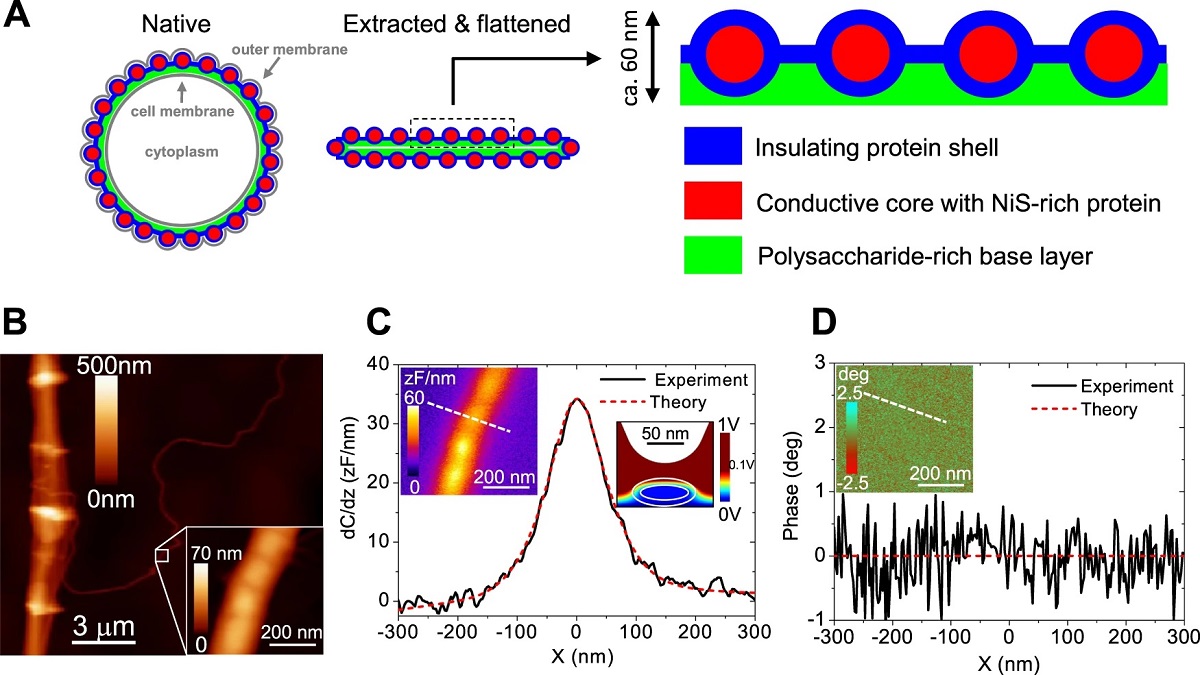
Figure 5 from Henricus T. S. Boschker et al. “Efficient long-range conduction in cable bacteria through nickel protein wires”
A Compositional model of the conductive fiber sheath in cable bacteria based on the present findings. Cross-sections through a filament in the middle of a cell are drawn and the number of fibers has been reduced for clarity—a 4 μm diameter cable bacterium has typically ~60 fibers5. In its native state (right panel), the fiber sheath is embedded periplasm between the cell and outer membrane and adopts a circular shape. After extraction, which removes the membranes and most of the cytoplasm and after drying upon a surface for analysis, the fiber sheath flattens, leading to two mirrored sheaths on top of each other (middle panel). The enlargement shows a section of the top sheath, which is the sample section probed by ToF-SIMS depth profiles and NanoSIMS images. Fibers are made of protein with a conductive Ni/S rich core and a non-conductive outer shell, and are embedded in a basal layer enriched in polysaccharide. B Topographic AFM image of a fiber sheath with a single isolated fiber detaching. The insert shows a detailed AFM image of this single fiber. C SDM amplitude image (right insert) and cross-sectional profile. D Corresponding SDM phase image (insert) and cross-sectional profile. Constant height (z = 66 nm) cross-section profiles are measured along the dashed lines shown in the left inserts. The red dotted lines in C and D represent model fits assuming the a fiber has a conductive core and an insulating outer shell. The right insert in C shows a vertical cross-section of the electric potential distribution as predicted by the model. Model parameters: shell thickness, d = 12 nm; fiber height, h = 42 nm; fiber width w = 87 nm; relative dielectric constants of the shell and core, εs = ω εc = 3; conductivity of the shell σs = 0 S/cm (insulating); conductivity of the core σc = 20 S/cm7 (see Supplementary Note 2 for treatment of SDM results and models tested). SDM analysis on a single fiber is available only from one samples as this is a rare event, but results from a double fiber and fiber sheaths are in agreement (see Supplementary Note 2).
*Henricus T. S. Boschker, Perran L. M. Cook, Lubos Polerecky, Raghavendran Thiruvallur Eachambadi, Helena Lozano, Silvia Hidalgo-Martinez, Dmitry Khalenkow, Valentina Spampinato, Nathalie Claes, Paromita Kundu, Da Wang, Sara Bals, Karina K. Sand, Francesca Cavezza, Tom Hauffman, Jesper Tataru Bjerg, Andre G. Skirtach, Kamila Kochan, Merrilyn McKee, Bayden Wood, Diana Bedolla, Alessandra Gianoncelli, Nicole M. J. Geerlings, Nani Van Gerven, Han Remaut, Jeanine S. Geelhoed, Ruben Millan-Solsona, Laura Fumagalli, Lars Peter Nielsen, Alexis Franquet, Jean V. Manca, Gabriel Gomila and Filip J. R. Meysman
Efficient long-range conduction in cable bacteria through nickel protein wires
Nature Communications volume 12, Article number: 3996 (2021)
DOI: https://doi.org/10.1038/s41467-021-24312-4
Please follow this external link to read the full article:
Open Access: The article “Efficient long-range conduction in cable bacteria through nickel protein wires” by Henricus T. S. Boschker, Perran L. M. Cook, Lubos Polerecky, Raghavendran Thiruvallur Eachambadi, Helena Lozano, Silvia Hidalgo-Martinez, Dmitry Khalenkow, Valentina Spampinato, Nathalie Claes, Paromita Kundu, Da Wang, Sara Bals, Karina K. Sand, Francesca Cavezza, Tom Hauffman, Jesper Tataru Bjerg, Andre G. Skirtach, Kamila Kochan, Merrilyn McKee, Bayden Wood, Diana Bedolla, Alessandra Gianoncelli, Nicole M. J. Geerlings, Nani Van Gerven, Han Remaut, Jeanine S. Geelhoed, Ruben Millan-Solsona, Laura Fumagalli, Lars Peter Nielsen, Alexis Franquet, Jean V. Manca, Gabriel Gomila & Filip J. R. Meysman is licensed under a Creative Commons Attribution 4.0 International License, which permits use, sharing, adaptation, distribution and reproduction in any medium or format, as long as you give appropriate credit to the original author(s) and the source, provide a link to the Creative Commons license, and indicate if changes were made. The images or other third party material in this article are included in the article’s Creative Commons licence, unless indicated otherwise in a credit line to the material. If material is not included in the article’s Creative Commons licence and your intended use is not permitted by statutory regulation or exceeds the permitted use, you will need to obtain permission directly from the copyright holder. To view a copy of this licence, visit http://creativecommons.org/licenses/by/4.0/.