The idea of using virus-like particles as nanocarriers for heterologous cargo transport and delivery requires controlling the stability of the container–cargo system.*
In particular, the conditions of the cargo delivery entail tailoring the escape of the molecular payload when the virus particle is disassembled. What happens to the internalized molecules when the nanocage is opened? *
To this end, it is necessary to control the cargo–container interaction which, in turn, would tune the retention of cargo when the disassembly of the nanocarrier takes place. Thus, it is necessary to develop nanocarrier systems that facilitate the control of the cargo retention conditions as a function of its interaction with the nano-container.*
In the article “Mechanical disassembly of human picobirnavirus like particles indicates that cargo retention is tuned by the RNA–coat protein interaction” María J. Rodríguez-Espinosa, Javier M. Rodríguez, José R. Castón and Pedro J. de Pablo describe how they designed three mutants of human picobirnavirus where the RNA–coat protein interaction, observed previously via cryo-electron microscopy, is modified by changing the N-terminal end of the coat protein.
The authors used atomic force microscopy (AFM) for inducing the mechanical unpacking of the RNA internalized in particles of each mutant.*
Their experiments crack-opened individual particles in real time to monitor the cargo release. Among other results, María J. Rodríguez-Espinosa et al. have measured that an increment in the N-terminal length by just 8% increases the cargo retention of partially disrupted particles by a factor of 10 with respect to the wild type. *
This study elucidates the interplay between the RNA–coat protein interaction of each mutant and their capacity for cargo retention during disassembly.*
For mechanical fatigue experiments, rectangular AFM cantilevers (NANOSENSORS™, uniqprobe qp-BioAC AFM probes with three different AFM cantilevers per chip) with nominal spring constants of 0.05 and 0.1 N m−1 were used.
The small variation of AFM cantilever force constant and resonance frequency of all types of the uniqprobe AFM probes series makes them the ideal AFM probes for biology and life science applications.
The AFM cantilevers were calibrated using Sader’s method.
Mechanical fatigue experiments were carried out on single particles, taking consecutive images at a low and constant force of ∼50 pN, below its breaking force. Images were processed and analyzed frame-by-frame using WSxM software39 to obtain additional information about the topography transformation of virus particles during the experiments. The mechanical fatigue experiments were performed in 10 Δ45-CP, 18 CP VLPs and 10 Ht-CP VLPs. Enough frames were obtained until the disruption of the particles took place. The variations over time of the VLPs area and height increments were monitored from the initial value 0 for each frame (Fig. 5).*
In mechanical fatigue experiments, the AFM tip erodes the VLPs gradually while imaging (Fig. 5(A) and Movies SM1–SM3, ESI† in the cited article), removing the virus material which can be either adhered by the surface or diffused to the liquid environment. Monitoring the area covered with debris for each hPBV variant reveals that RNA helps to keep the capsomer debris attached to the HOPG surface after being lost from the capsid during the mechanical fatigue procedure (Fig. 5(B) in the cited article).*
Fig. 5 from María J. Rodríguez-Espinosa et al. 2023:
Analysis of individual virus particles unpacking under mechanical fatigue.
(A) Evolution of individual particles under mechanical fatigue for the three variants: Δ45-CP (left), CP (center) and Ht-CP (right). Scale bar: 25 nm. The initial frame shows the intact particle, the intermediate frame reveals some damage and the last frame is the image of the final collapsed state. The entire fatigue process can be visualized in Movies SM1, SM2 and SM3 (ESI†) for Δ45-CP, CP and Ht-CP, respectively. The color of the height scale is the same as shown in Fig. 2. (B) Evolution of the particle surrounding the covered area with debris for each VLP: 10 Δ45-CP VLPs (left), 18 CP VLPs (center) and 10 Ht-CP VLPs (right). (C) Evolution of the particle height for each virus particle, grouped by the hPBV variant. The dotted dark lines indicate the rate of collapse (RoC) ratio fitting for three cases in Δ45-CP (left, −7 nm per frame), CP (center, −6 nm per frame) and Ht-CP (right, −0.5 nm per frame), see the text. (D) Lost volume for each virus particle, classified by the hPBV variant. The dotted dark lines indicate the RoC ratio fitting for three cases in Δ45-CP (left, −21 000 nm3 per frame), CP (center, −21 000 nm3 per frame) and Ht-CP (right, −2000 nm3 per frame), see the text. The elapsed time for 1 frame is 120 seconds, resulting in 2 hours 30 minutes for the 80 frames of the x axes.
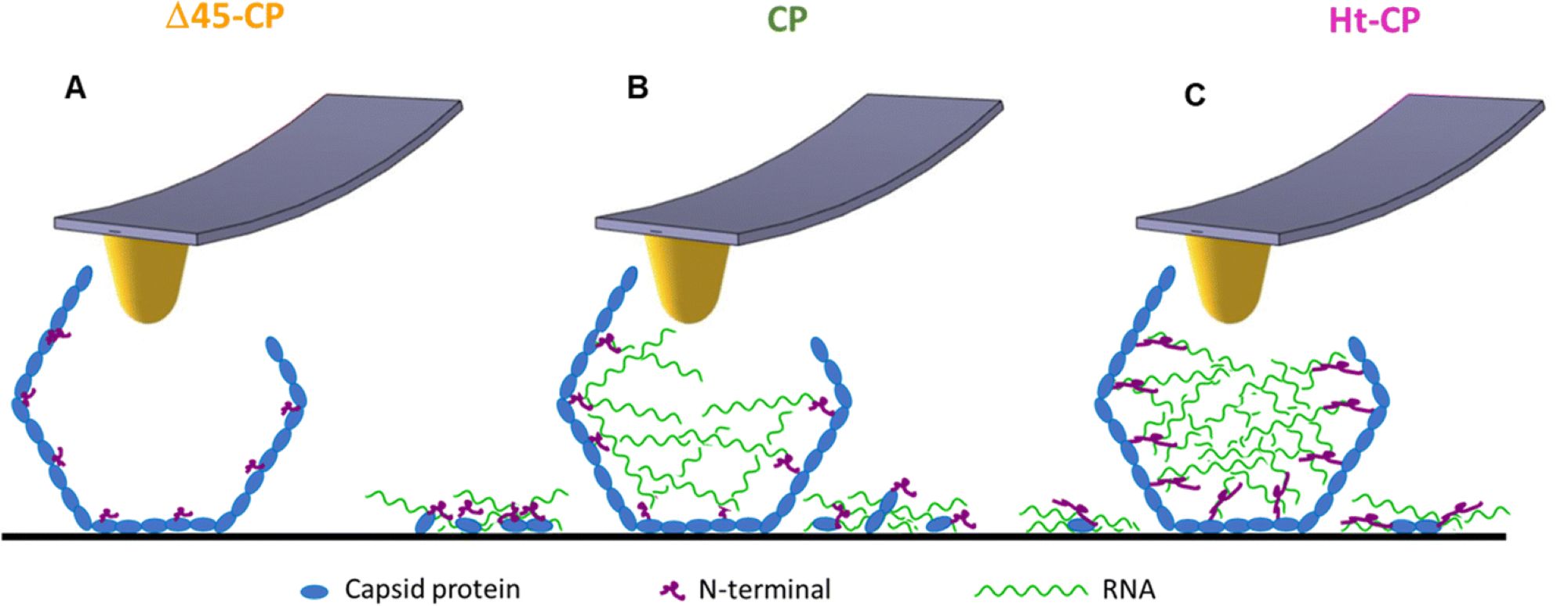
Fig. 6 from María J. Rodríguez-Espinosa et al. 2023:
Model depicting the RNA cargo retention for each VLP variant. The Δ45-CP VLP structure (A) does not show externalized cargo. The CP VLP structure (B) shows shorter N-terminals compared to the Ht-CP structure (C), which implies less RNA cargo retention compared to the Ht-CP structure. The cartoons in blue, green and pink colors represent coat protein, RNA and N-terminal, respectively.
*María J. Rodríguez-Espinosa, Javier M. Rodríguez, José R. Castón and Pedro J. de Pablo
Mechanical disassembly of human picobirnavirus like particles indicates that cargo retention is tuned by the RNA–coat protein interaction
Nanoscale Horizons, 2023, 8, 1665-1676
DOI: https://doi.org/10.1039/D3NH00195D
The article “Mechanical disassembly of human picobirnavirus like particles indicates that cargo retention is tuned by the RNA–coat protein interaction” by María J. Rodríguez-Espinosa, Javier M. Rodríguez, José R. Castón and Pedro J. de Pablo is licensed under a Creative Commons Attribution 3.0 International License, which permits use, sharing, adaptation, distribution and reproduction in any medium or format, as long as you give appropriate credit to the original author(s) and the source, provide a link to the Creative Commons license, and indicate if changes were made. The images or other third-party material in this article are included in the article’s Creative Commons license, unless indicated otherwise in a credit line to the material. If material is not included in the article’s Creative Commons license and your intended use is not permitted by statutory regulation or exceeds the permitted use, you will need to obtain permission directly from the copyright holder. To view a copy of this license, visit https://creativecommons.org/licenses/by/3.0/.